By Paolo Rega
In a groundbreaking advance for genetic engineering and immunotherapy, researchers at the Karolinska Institutet have unveiled a novel optogenetic CRISPR system that enables precise, light-controlled gene editing in T lymphocytes — even inside living organisms. The system, dubbed BLU-VIPR (Blue Light-inducible Universal VPR-Improved Production of RGRs), allows scientists to turn gene editing on or off with blue light, effectively handing them a remote control for precise genetic manipulation.
The study, recently published in Nucleic Acids Research, offers not only a powerful new tool for basic science but also a future path for treating diseases like cancer through more targeted, less invasive gene therapies.
CRISPR Meets Light
CRISPR has revolutionized gene editing, allowing scientists to snip and tweak DNA with remarkable precision. But controlling when and where genes are edited remains a critical challenge — especially in living animals. Traditional CRISPR systems are always “on” once delivered, which can lead to off-target effects and complicate the study of dynamic biological processes.
Enter optogenetics, a technique that uses light to control cells. In neuroscience, optogenetics has already transformed how researchers study the brain by activating neurons with lasers. The Karolinska team set out to merge this concept with gene editing.
From Protein Focus to gRNA Control
What makes BLU-VIPR stand apart is its focus on regulating the guide RNA (gRNA) — not the Cas9 protein, which does the actual DNA cutting.
Most previous optogenetic CRISPR systems rely on splitting the Cas9 enzyme and reassembling it with light. While clever, these systems are bulky and difficult to deliver into living cells, especially the hard-to-edit T lymphocytes, which are crucial in immune responses and immunotherapy.
Instead, the researchers engineered a light-sensitive transcription factor called VPR-EL222. When exposed to blue light, VPR-EL222 switches on transcription of a custom-made RNA transcript that includes both a reporter protein (like mCherry) and the gRNA. Cleverly, the gRNA is flanked by self-cleaving ribozymes that snip it free once the transcript is made — a design known as RGR (ribozyme-gRNA-ribozyme).
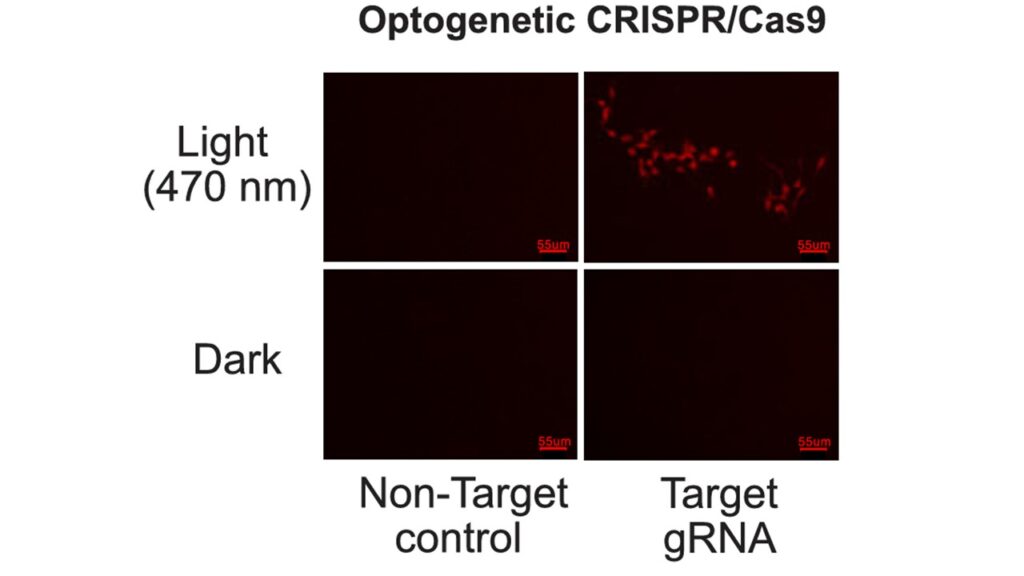
Turning Light into Edits: Proof of Concept
To test their invention, the team started with HEK293T cells and used blue light (470 nm) to activate gene editing. They designed a reporter system where a gene called tdTomato would only fluoresce if the DNA was corrected by Cas9. The results were striking: after illumination, cells began to glow red, confirming successful, light-controlled editing.
But they didn’t stop there. The researchers demonstrated that BLU-VIPR could perform not only standard indels (insertions/deletions), but also CRISPR activation (CRISPRa) and base editing — where single DNA letters (A, C, T, G) are chemically swapped.
Multiplexing and Modularity
Another major advantage of BLU-VIPR is its modularity. By embedding multiple RGR units in a single transcript, the system can simultaneously express several gRNAs targeting different genes. Even more impressively, they showed orthogonal activation using two different Cas effectors — dCas9-VPR and dCas12a-VPR — controlled by distinct gRNAs from the same light-inducible message.
The Real Test: T Cells in Living Mice
Perhaps the most exciting part of the study came when the team turned their attention to T lymphocytes, the immune system’s elite soldiers. These cells are notoriously difficult to genetically modify, particularly with optogenetic tools that require large payloads.
Using retroviruses, the team delivered BLU-VIPR constructs to primary T cells isolated from Cas9-expressing mice. After blue light exposure, they saw effective gene knockout of Thy1.2, a surface marker. Importantly, no editing occurred without light — demonstrating exquisite control.
Then came the ultimate test: in vivo optogenetic editing. In a surgical setup adapted from intravital microscopy, the researchers exposed a single lymph node in live mice to blue light for just one hour. Within 48 hours, they detected gene editing in T cells at that precise location — nowhere else.
Implications: A Toolkit for Precision Immunotherapy
While this is still early-stage research, the implications are vast.
In the future, cancer immunotherapies like CAR-T cells could be engineered with BLU-VIPR to activate or silence genes only when needed — for example, inside a tumor, or after detecting a specific signal. The platform also holds promise for neuroscience, developmental biology, and any field that benefits from spatiotemporal gene control.
However, with any technology, challenges remain. BLU-VIPR requires blue light penetration, which is limited in deep tissues. But researchers are already exploring red-shifted optogenetic systems, and combining them with evolved AAV vectors for better delivery. Furthermore, while BLU-VIPR depends on Cas proteins being present in the cells, this is increasingly achievable with transgenic models or co-delivery techniques.
Leave a Reply