Understanding why individuals of the same chronological age often have different health outcomes has long puzzled scientists. Biological age, which reflects a person’s physiological state, offers a more precise measure of aging than chronological age. A recent study titled “Cell-type specific epigenetic clocks to quantify biological age at cell-type resolution“ introduces a transformative tool to measure biological age at the cellular level. This innovation, called cell-type-specific epigenetic clocks, opens new avenues for studying aging and age-related diseases.
Epigenetic Clocks: A Limit of Precision
Epigenetic clocks estimate biological age by analyzing DNA methylation, chemical changes that regulate gene expression. While these clocks have been revolutionary, they often use data from bulk tissue samples, blending signals from various cell types. This approach fails to account for differences in how individual cells age, limiting the clocks’ ability to detect subtle changes associated with specific diseases.
For example, shifts in lymphocyte populations contribute to aging in blood, while changes in neuronal and glial cells affect aging in the brain. Traditional clocks provide composite readings, which obscure these cell-specific dynamics. This limitation prevents researchers from understanding which cells drive age-related diseases or respond best to treatments.
A New Approach to Aging
The study introduces cell-type-specific epigenetic clocks, which measure biological age at the cellular level. These clocks separate two components of aging: intrinsic aging, driven by DNA methylation changes within cells, and extrinsic aging, reflecting shifts in cell-type composition within tissues. The researchers found that extrinsic aging accounted for 39% of an epigenetic clock’s accuracy in blood and 12% in the brain. By isolating these components, the new clocks provide a clearer picture of cellular aging.
Case Studies: Brain and Liver Clocks
The study applied these clocks to neurons, glial cells, and hepatocytes (liver cells), yielding groundbreaking results. Neuron- and glia-specific clocks revealed accelerated biological aging in Alzheimer’s disease (AD). The most significant age acceleration occurred in glial cells within the temporal lobe, suggesting that these cells play a key role in AD progression. Intriguingly, neuronal aging in AD appeared to stem from changes in DNA methylation rather than shifts in cell populations, providing new insights into neurodegeneration.
In liver tissue, the hepatocyte-specific clock detected accelerated aging in conditions such as non-alcoholic fatty liver disease (NAFLD) and obesity. Unlike traditional liver clocks, the hepatocyte clock captured subtle cellular changes, demonstrating its superior sensitivity. These findings highlight the potential of cell-type-specific clocks to uncover disease mechanisms and guide targeted interventions.
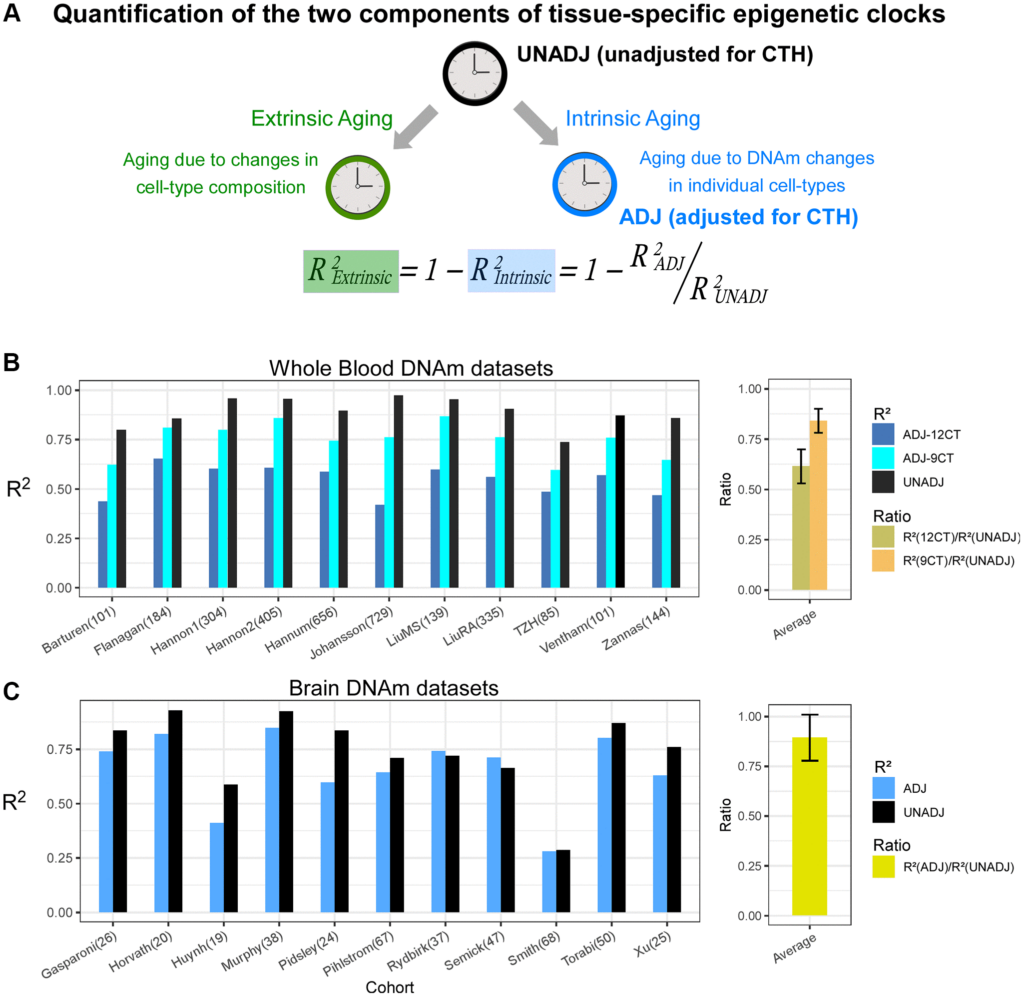
Molecular Insights and Disease Pathways
The clocks also shed light on the molecular drivers of aging. Several DNA methylation sites used in the clocks overlapped with genes implicated in neurodegenerative diseases and liver pathologies. For example, genes such as SESN2 and TOMM40L, associated with Alzheimer’s disease, were linked to the neuron- and glia-specific clocks. This overlap suggests that the clocks capture not only biological age but also disease-relevant molecular changes, making them valuable tools for research and therapy development.
Implications for Aging Research
The introduction of cell-type-specific clocks marks a paradigm shift in aging research. These tools enable scientists to:
- Detect Early Disease: By identifying accelerated aging in specific cell types, the clocks can serve as early indicators of conditions like Alzheimer’s or liver disease.
- Develop Targeted Therapies: Understanding which cells age faster allows for treatments that focus on the most vulnerable populations.
- Personalize Medicine: Tailoring interventions to an individual’s cellular aging profile could improve health outcomes and longevity.
Challenges and Future Directions
While promising, the approach has limitations. Building these clocks requires large datasets to ensure accuracy, especially for complex tissues like the brain. Expanding the clocks to include more cell types and higher resolution will require collaboration and integration of global datasets. Additionally, exploring the interaction between genetic and environmental factors will help refine the clocks further.
Conclusion
Cell-type-specific epigenetic clocks offer an unprecedented view into the biology of aging. By measuring biological age at the cellular level, they provide insights into how different cells contribute to aging and age-related diseases. This innovation not only advances our understanding of aging but also lays the groundwork for early detection and personalized interventions, paving the way for healthier, longer lives.