In a groundbreaking paper published in Science, a team of scientists from the California Institute of Technology (Caltech) has unveiled a remarkable achievement in the realm of enzymatic evolution. Titled “Directed evolution of enzymatic silicon-carbon bond cleavage in siloxanes,” the research introduces a novel approach to manipulating bacterial cytochrome P450 variants for breaking silicon-carbon bonds in volatile methylsiloxanes (VMS), a prominent subgroup of the siloxane family.
Innovative Evolution to Engineer Cytochrome P450
Employing the innovative technique of artificial evolution, the scientists, led by Frances Arnold, PhD, the Linus Pauling Professor of Chemical Engineering, Bioengineering, and Biochemistry at Caltech, aimed to engineer a cytochrome P450 variant capable of efficiently cleaving silicon-carbon bonds in both linear and cyclic VMS. This method not only opens doors to the creation of industrially valuable products but also addresses the environmental concerns associated with VMS, known for their potential as pollutants.
The researchers initiated the project by identifying a variant of cytochrome P450 from their enzyme collection with a modest ability to break silicon-carbon bonds. This served as the starting point for the evolution of Si-C bond cleavage activity. Through a series of mutations to the enzyme’s DNA, the team systematically tested new variants, with the most promising performers undergoing further mutations in subsequent rounds of testing. The iterative process ultimately led to the development of an enzyme demonstrating significant activity in breaking silicon-carbon bonds, enabling a comprehensive study of the reaction’s products and mechanism.

Implications Beyond Enzymatic Engineering
Frances Arnold, a pioneer in directed evolution and the recipient of the 2018 Nobel Prize in Chemistry for her groundbreaking work, expressed the broader implications of the research, stating, “For example, natural organisms could evolve in siloxane-rich environments to catalyze a similar reaction, or further improved versions of laboratory-evolved enzymes such as this one could possibly be used to treat siloxane contaminants in wastewater.”
The significance of this research extends beyond its applications in enzymatic engineering. Siloxanes, with their unique material properties stemming from the silicon-oxygen backbone and silicon-methyl groups, have both inorganic and organic characteristics. Dr. Dimitris (Dimi) Katsoulis of Michigan-based Dow Inc., key contributor to the study, highlighted, “The silicon–oxygen backbone gives the polymer an inorganic-like character while the silicon–methyl groups give the polymer organic-like characteristics. Thus, these polymers have unique material properties, such as high thermal and oxidative stability, low surface tension, and high backbone flexibility among others.”
Parallel Research
In a parallel study titled “A bacterium that degrades and assimilates poly(ethylene terephthalate),” another group of researchers explored the realm of plastic degradation through a polyethylene terephthalate (PET)-degrading enzyme. Discovered in the bacteria Ideonella sakaiensis in 2016, this enzyme has sparked interest in the possibility of harnessing biological agents to address the global plastic pollution crisis. The study reflects a collective effort to leverage the power of enzymatic evolution for environmentally significant applications.
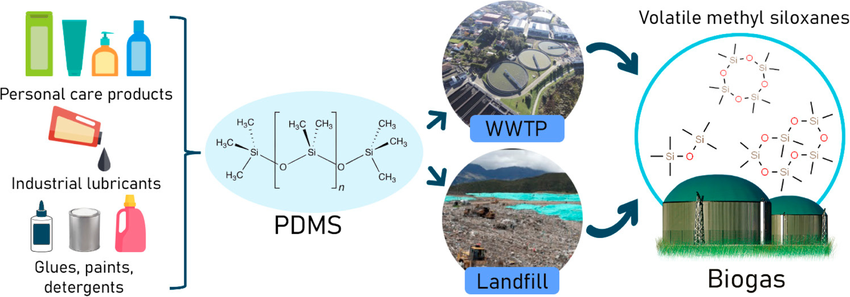
The convergence of these studies highlights the versatility of artificial evolution as a tool for addressing environmental challenges and unlocking the potential of enzymes. As researchers continue to delve into the intricacies of directed evolution, the scientific community stands poised to witness further breakthroughs with far-reaching implications for industry and environmental sustainability.