In the battle against infectious diseases, antibiotic resistance remains one of the most pressing global health threats. A recent study published in Nature Communications sheds light on a crucial driver of multidrug resistance (MDR) in bacteria: the hypermutation caused by DNA mismatch repair (MMR) deficiency. The research, focusing on Pseudomonas aeruginosa, underscores the role of mutational signature analysis in predicting bacterial hypermutation and, consequently, MDR. This discovery holds promise for precision medicine, offering a novel diagnostic tool to guide targeted antibiotic therapies.
Understanding Bacterial Hypermutation
Bacteria such as Pseudomonas aeruginosa can become hypermutators when they lose their DNA mismatch repair (MMR) system. MMR is crucial in correcting replication errors, and its deficiency leads to an increased mutation rate. This phenomenon accelerates the bacteria’s ability to develop resistance to antibiotics, making infections harder to treat.
Hypermutators are often found in clinical settings, particularly in chronic respiratory infections. Notably, up to 60% of P. aeruginosa isolates from patients with cystic fibrosis (CF) exhibit MMR deficiency. While MMR deficiency is associated with rapid resistance to single antibiotics, its role in driving multidrug resistance acquisition remained unclear—until now.
The Study: How MMR Deficiency Fuels Resistance
The researchers sought to determine how MMR deficiency contributes to MDR in P. aeruginosa. Using in vitro adaptive evolution, they exposed MMR-deficient strains of the bacteria to first-line and last-resort antibiotics. Whole genome sequencing (WGS) was performed to analyze the mutational signature associated with MMR deficiency and track resistance development.
Their findings revealed that hypermutated P. aeruginosa acquired MDR regardless of antibiotic class. Instead of developing resistance through drug-specific mechanisms, the bacteria relied on common resistance pathways shared between different drugs. This suggests that traditional monotherapy, where one antibiotic is used at a time, may inadvertently accelerate MDR in hypermutator strains.
The Role of Mutational Signature Analysis
A key takeaway from the study is the potential of mutational signature analysis as a predictive tool. By examining mutational patterns in clinical isolates of P. aeruginosa, researchers identified bacteria that were already MDR or highly prone to acquiring MDR in the future. These findings suggest that mutational signature analysis could be used as a diagnostic approach to identify high-risk bacterial strains before they become untreatable.
The ability to predict whether a bacterial strain is likely to develop multidrug resistance is a crucial step forward. It means clinicians can intervene earlier with targeted treatments rather than waiting for standard antibiotic therapies to fail.
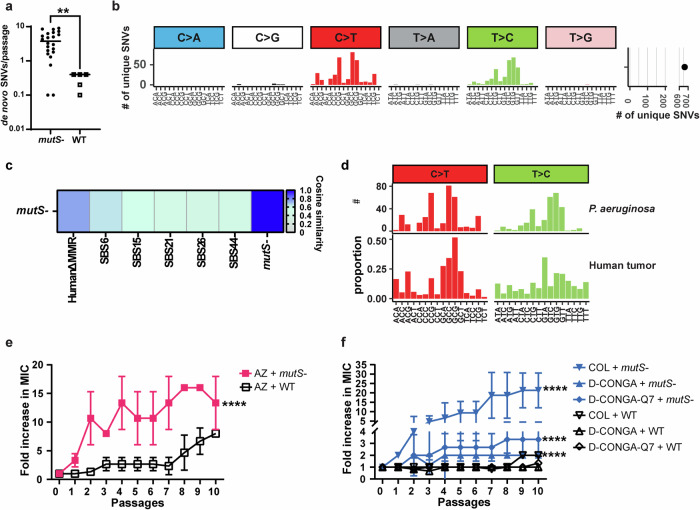
Preventing Multidrug Resistance: A New Strategy
One of the study’s most promising insights is the discovery that rational combination therapies can prevent MDR acquisition in hypermutator P. aeruginosa. The researchers found that combining antibiotics with different mechanisms of action—such as pairing a traditional antibiotic with an antimicrobial peptide—significantly reduced the bacteria’s ability to develop resistance.
This contrasts with conventional cycling of antibiotics, which often involves alternating between two drugs. The study found that such cycling strategies could actually promote resistance rather than curb it. This finding is particularly relevant for CF patients, where P. aeruginosa infections are commonly treated with inhaled antibiotics in a cycled regimen.
By strategically combining drugs that require different resistance mechanisms, it is possible to reduce the likelihood of bacteria developing multidrug resistance.
Beyond Cystic Fibrosis: Wider Implications
While the study primarily focused on P. aeruginosa in CF patients, its implications extend far beyond. Analysis of publicly available bacterial genomes revealed that MMR-deficient strains are also present in other infection contexts, including respiratory tract infections, urinary tract infections, and intra-abdominal infections. This suggests that MMR deficiency may play a broader role in antibiotic resistance across different bacterial infections.
Further, the findings raise concerns about how standard antibiotic treatment strategies might inadvertently select for hypermutator strains. As antibiotic resistance continues to escalate globally, understanding the genetic and evolutionary mechanisms behind MDR acquisition is critical to developing effective countermeasures.
A Step Towards Precision Medicine
The study’s results align with a growing trend in medicine—leveraging genomic data for personalized treatment approaches. If implemented in clinical practice, mutational signature analysis could help healthcare providers identify MDR-prone infections early, allowing for tailored treatment plans that minimize resistance development.
Currently, WGS is becoming more accessible for clinical use, paving the way for real-time mutational signature analysis in hospitals. By integrating this technology with patient diagnostics, clinicians could move away from reactive antibiotic treatment and towards proactive, precision-based care.
Looking Ahead
As the fight against antibiotic resistance intensifies, the findings from this study highlight the importance of genetic surveillance in bacterial infections. Future research should focus on expanding mutational signature analysis to other bacterial pathogens and exploring its potential in real-time diagnostic settings.
This research marks a step in the right direction. By identifying high-risk bacterial strains early, treatment strategies can be refined to counteract resistance before it becomes an insurmountable challenge. In the face of an evolving antibiotic resistance crisis, innovation in diagnostic tools and treatment approaches is more critical than ever. Mutational signature analysis offers a promising path toward precision medicine, ensuring that life-saving antibiotics remain effective for generations to come.